Activation of RhoA/ROCK2 signaling by hypoxia-inducible factor 1α in promoting tumor growth and metastasis in human colon cancer
Highlight box
Key findings
• Hypoxia-inducible factor 1α (HIF-1α) promotes colon carcinoma progression through the RhoA/ROCK2 signaling pathway.
What is known and what is new?
• Hypoxia is one of the most common features of the pathological development of colon carcinoma.
• This study confirmed a regulatory relationship between HIF-1α and the RhoA/ROCK2 signaling pathway in colon cancer.
What is the implication, and what should change now?
• This study has identified a new molecular regulatory mechanism for the occurrence and development of colon cancer, providing a new target for the treatment of this disease.
Introduction
Colorectal cancer is one of the most common cancers in the world and is the leading cause of cancer-related death in many countries (1), accounting for about 10% of all cancers and cancer-related deaths diagnosed each year worldwide. In developing countries, the incidence of colorectal cancer will continue to increase (2). Most patients with colorectal cancer seek treatment due to clinical symptoms, but patients who have reached the middle or late stages are beyond the optimal treatment period and thus have a poor prognosis (3). However, the pathogenesis of colorectal cancer is complex, and so research directed toward clarifying the molecular mechanism of colon cancer pathogenesis and progression is needed.
Hypoxia is one of the most common features of the pathological development of diseases, including cardiovascular, inflammatory, infectious, and neoplastic diseases (4,5). Hypoxia can cause a series of pathological changes, such as abnormal angiogenesis, metabolic shift, and immunosuppression of tumors, which shape the tumor microenvironment (TME) into a niche that promotes tumor progression and metastasis, thus reducing the effectiveness of antitumor therapy or promoting drug resistance (6). With the discovery of oxygen-sensing signaling, numerous molecular pathways and biological processes associated with hypoxia have been discovered and elucidated (7). It has been found that the cellular response to hypoxia is achieved through a signaling pathway mediated by hypoxia inducible factor (HIF). HIF regulates the expression and function of more than 1,000 genes in human body, including glucose transporter, vascular endothelial growth factor (VEGF), erythropoietin (EPO), etc. (4,8). There are three subtypes of HIF, including HIF-1α, HIF-2α, and HIF-3α, each of which exerts different functions in the body (5,9-11). HIF-1α, one of the most widely studied hypoxic dimers, is activated in response to hypoxic exposure, promoting adaptability to the environment and determining tolerance to hypoxia (12). Expression levels of HIF-1α are commonly detected in various types of cancer tissue (13). Abnormal HIF-1α overexpression is associated with cell survival, epithelial-mesenchymal transition, and cancer metastasis (14). A study has found that the expression of HIF-1α is closely related to the invasion and metastasis of liver cancer; for instance, the high expression of HIF-1α often leads to the poor prognosis of patients with hepatocellular carcinoma and thus may be a key target for inhibiting the metastasis of this disease (15).
Ras homolog family member A (RhoA) is a small molecule of G protein that cycles between active [guanosine triphosphate (GTP) binding] and inactive [guanosine diphosphate (GDP) binding] forms. GTP-RhoA is involved in the regulation of cellular function through its interaction with downstream Rho-associated coiled-coil protein kinase (ROCK) (16). ROCK is a serine-threonine protein kinase that contains the 2 isomers: ROCK1 and ROCK2 (17). RhoA/ROCK is involved in a variety of physiological processes, such as actin cytoskeletal recombination, cell growth control, chemotaxis, and morphological change, and by regulating the activation of p38 MAPK, these proteins are also involved in inflammation (16,18). However, HIF-1α and RhoA/ROCK2 have not been extensively investigated in colorectal cancer, and their mechanisms of action remain unclear. Further exploration of the in-depth molecular mechanism can provide new insights into the progression mechanism of colorectal cancer and provide new targets and directions for the treatment of clinical colorectal cancer.
In this study, we aimed to determine the expression of HIF-1α and RhoA/ROCK2 in colorectal cancer tissues. Through cellular experiments, we were able to characterize the regulatory relationship between HIF-1α and the RhoA/ROCK2 signaling pathway and to clarify the molecular mechanism by which HIF-1α promotes colorectal cancer progression through the RhoA/ROCK2 signaling pathway. We present this article in accordance with the MDAR reporting checklist (available at https://jgo.amegroups.com/article/view/10.21037/jgo-23-844/rc).
Methods
Human tissue samples
Colon cancer tissue was collected with approval from the Ethics Committee of the First Affiliated Hospital of Chongqing Medical University (Chongqing, China) (Approval No. 20150612). The study was conducted in accordance with the Declaration of Helsinki (as revised in 2013). Informed consent was taken from all the patients. Specifically, colon cancer and corresponding noncancerous tissues were collected from patients who had undergone radical colectomy but not preoperative chemoradiotherapy, these tissues are based at the First Affiliated Hospital of Chongqing Medical University. The histologic type of the tissue samples was independently evaluated by two pathologists, and the detailed information of all patients was recorded.
Cell lines and cell culture
The SW480, SW620, HCT116, and HT29 cell lines were purchased from the Shanghai Cell Bank at the Chinese Academy of Sciences (Shanghai, China). All of the cells were cultured in RPMI-1640 medium (HyClone, Logan, UT, USA) supplemented with 10% fetal bovine serum (HyClone) and maintained at 37 ℃ in a 5% CO2 humidified atmosphere. All cell lines were authenticated by short tandem repeat (STR) profiling and all experiments were performed with mycoplasma-free cells. Hypoxia culture was performed in a three-chamber air incubator flushed with a gas mixture of 5% CO2 and 94% N2 at 37 ℃. The final O2 pressure of the medium was measured at a range of 0.5–1%. The above method is widely used to induce hypoxic stress.
Adenovirus-mediated small interfering RNA (siRNA) targeting HIF-1α
The adenovirus vector was designed, constructed, packed, and purified by HanBio Therapeutics (Shanghai, China) and was processed according the manufacturer’s protocol. The sequence of interference was as follows: 5'-GGAAATGAGAGAAATGCTTAC-3'. We performed siRNA transfection using SW480 and SW620 cell lines.
Lentivirus-mediated overexpression of RhoA
The lentivirus targeting RhoA and an empty vector were designed, packed, and purified by GeneChem (Shanghai, China) and used according to the protocol provided by the manufacturer. We performed LV-RNA transfection using SW480 and SW620 cell lines.
Immunohistochemical (IHC) analysis
Fresh tissue samples were divided into two parts, with one part being used for IHC assay and hematoxylin and eosin (HE) staining and the other being used for an array of subsequent experiments. Sections (4-µm thick) of colon cancer specimens and their corresponding noncancerous specimens were cut from paraffin-embedded wax blocks. Standard immunostaining was performed using an SP9000 Immunohistochemistry Kit (Zhongshan Golden Bridge, Beijing, China) according to the manufacturer’s instructions and was followed by incubation with the primary antibodies for HIF-1α (1:200; ab228649, Abcam, Cambridge, MA, USA), RhoA (1:150; GTX66647, Gene Tex, California, USA), and ROCK2 (1:200; ab66320, Abcam, Cambridge, MA, USA). After immunostaining, all target proteins were independently and blindly analyzed by two pathologists using a transmission light microscope.
Quantitative real-time polymerase chain reaction (qRT-PCR)
Total RNA was extracted from tissues and cells using TRIzol reagent (Takara Bio, Kusatsu, Japan). After reverse transcriptional reaction, qRT-PCR was performed with a CFX9 Real-Time System (Bio-Rad Laboratories, Hercules, CA, USA) and SYBR Premix Ex TaqII (Takara Bio). GAPDH is used as an internal reference gene. Analyze the data according to the 2-ΔΔCt method. The primers for HIF-1α, RhoA, and ROCK2 were as follows: HIF-1α: forward, 5'-CCA CAG AAA CTA CCT TCA ACT CC-3', and reverse, 5'-GTG ATC TCC TTC TGC ATC CTG T-3'; RhoA: forward, 5'-GCA GGT AGA GTT GGC TTT GTG G-3', and reverse, 5'-CGT TGG GAC AGA AAT GCT TGA C-3'; and ROCK2: forward, 5'-CGC CGT TGC CAT ATT AAG TGT-3', and reverse, 5'-CAC CAA CCG ACT AAC CCA CTT C-3'.
Western blotting
We used radioimmunoprecipitation with phenylmethylsulfonyl fluoride to extract cell and tissues proteins (Beyotime Institute of Biotechnology, Nanjing, China) method. The protein concentrations were measured using bicinchoninic acid (BCA) reagent (Beyotime Institute of Biotechnology) according to the manufacturer’s instructions. The protein was separated with sodium dodecyl sulfate-polyacrylamide gel electrophoresis (SDS-PAGE; 8–12% PAGE) and transferred onto a polyvinylidene fluoride (PVDF) membrane. It was then blocked with 5% skimmed milk powder at room temperature for 1 hour, after which primary antibodies were incubated overnight at 4 ℃, and secondary antibodies were incubated at 37 ℃ for 2 hours. The protein bands were visualized using an enhanced chemiluminescence (ECL) reagent (Beyotime Institute of Biotechnology) on a fusion imaging system (Proteinsimple, FluorChem R, California, USA).
Cell proliferation assay
Cell proliferation was measured using a Cell Counting Kit 8 (CCK8) reagent (Beyotime Institute of Biotechnology) according to the manufacturer’s instructions. Briefly, cells in the logarithmic growth phase were seeded in 96-well plates at densities of 2×103 cells per well. Cell proliferation was examined after 24 hours. Additionally, 10 µL of CCK8 was added to each well, and the cells were incubated at 37 ℃ for 2 hours. Finally, the absorbance was measured at 450 nm using a microplate reader (Multiskan Spectrum 1500, Thermo Fisher Scientific, Waltham, MA, USA).
Wound-healing assay
Cells were seeded in 6-well plates with RPMI-1640 medium for 24 hours and then scraped with an aseptic pipette tip. After a wash with phosphate-buffered saline (PBS), cells were incubated with completed RPMI-1640 for 24 hours. Cell migration images were observed and captured using a light microscope at 100× magnification.
Migration and invasion assay
The migration and invasion ability of cells were detected using the Transwell method, with 1×104 cells being seeded onto collagen-coated Transwell polyester membrane inserts with an 8-µm pore size in a 24-well plate. Complete RPMI (400 µL) was mixed into the upper chamber while serum-deprived RPMI (400 µL) was mixed into the lower chamber. After 24 hours in culture, cells were fixed in paraformaldehyde for 15 minutes and then stained with 0.05% crystal violet in PBS for 5 minutes. Cells on the underside of the filters were observed and counted under a light microscope.
Immunofluorescence assay
Immunofluorescence was performed to detect the coexpression of HIF-1α and RhoA in untransfected colon cancer cells. Cells were seeded and cultured on cover slips the day before analysis. After incubation for 24 hours, the cells were fixed by using 4% paraformaldehyde at 37 ℃ for 15 minutes. Then, 0.1% Triton was used for permeabilization at room temperature for 10 minutes. After a wash with PBS, cells were blocked in 5% bovine serum albumin (BSA) at 37 ℃ for 10 minutes and then incubated with HIF-1α and RhoA antibody at 4 ℃ overnight. After incubation with secondary antibodies [Alexa Fluor 488-conjugated goat anti-mouse immunoglobin G (IgG) and Alexa Fluor 594-conjugated goat anti-rabbit IgG] for 1 hours at 37 ℃, nuclei were counterstained with DAPI (Beyotime Institute of Biotechnology) for 5 minutes, and images were obtained using a fluorescence microscope (Olympus, Tokyo, Japan).
Statistical analysis
Statistical analysis was performed using SPSS 22.0 (IBM Corp., Armonk, NY, USA), Visualize the data using GraphPad Prism 5 (GraphPad Software, La Jolla, CA, USA). To compare two data groups, The t-test is used if the data meets the normal distribution, and the Mann-Whitney test is used if the data does not meet the normal distribution. The Kruskal-Wallis test was used to compare more than two groups. P<0.05 was considered statistically significant (19). All experiments in this study were repeated three times.
Results
HIF-1α, RhoA, and ROCK2 were highly expressed in human colon cancer
The IHC results showed that HIF-1α, RhoA, and ROCK2 exhibited stronger staining in colon cancer tissue than in corresponding noncancerous tissue (Figure 1A). In colon cancer tissue, the positive rate of HIF-1α, RhoA, and ROCK2 were 74%, 65%, and 68%, which were significantly higher than the 20%, 18%, and 27% in nonmalignant tissues. HIF-1α staining was located in the nucleus and cytoplasm, while RhoA and ROCK2 staining was mainly located in the cytoplasm. qRT-PCR results showed that the messenger RNA (mRNA) levels of HIF-1α, RhoA, and ROCK2 were significantly higher in malignant samples than in nonmalignant samples (Figure 1B). The protein level of HIF-1α, RhoA, and ROCK2 in the colon cancer tissues was confirmed to be definitively higher than that in normal colon tissues (Figure 1C). Western blotting indicated a higher protein expression of HIF-1α, RhoA, and ROCK2 in SW480 and SW620 as compared with HCT116 and HT29 (Figure 1D). We used SW480 and SW620 cell lines for follow-up experiments. In addition, the coexpression of HIF-1α and RhoA in untransfected colon cancer cells (SW480 and SW620) was detected by the means of immunofluorescence. HIF-1α (red) was mainly expressed in the nucleus and cytoplasm of colon cancer cells, while RhoA was expressed in the cytoplasm (Figure 2).
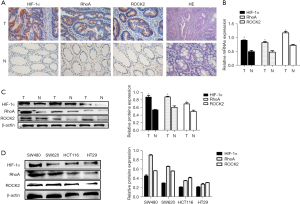
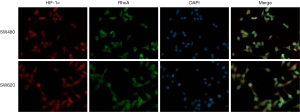
Knockdown of HIF-1α markedly suppressed colon cancer cell growth, migration, and invasion and inhibited RhoA/ROCK2 signaling pathway activation
In order to determine the effect of HIF-1α on the growth and metastasis of colon cancer cells, we used siRNA targeting HIF-1α to transfect the cells and decrease the expression of HIF-1α. Comparison with a control group revealed that HIF-1α expression was significantly decreased by siRNA group under normoxic conditions (Figure 3A) and hypoxic conditions (Figure 3B). The subsequent experiments were conducted under hypoxic conditions. Cell growth was reflected via cell viability assay using a CCK8 reagent, and the results showed that cells transfected with siRNA exhibited lower growth ability compared with control cells, with significant differences being found in the SW480 and SW620 cell lines in a hypoxic environment (Figure 4A). HIF-1α has been reported to play an important role in cell metastasis. In the wound-healing assay, the scratch in siRNA group remained mainly uncovered at 24 hours. However, the control cells had at least 70% coverage at 24 hours (Figure 4B). Moreover, the Transwell assay indicated that inhibition of HIF-1α could clearly reduce the invasion capability of SW480 and SW620 cells (Figure 4C). Moreover, we found that after knockdown of HIF-1α, the RhoA/ROCK2 pathway was inhibited, and the expressions of pMYPT1, cyclin D1, and MMP2 were significantly reduced in the siRNA group compared with the control group. These results indicate that knockdown of HIF-1α can significantly inhibit the proliferation, migration, and invasion of colon cancer cell lines SW480 and SW620 as well as the activation of RhoA/ROCK2 signaling pathway.
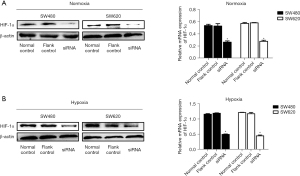
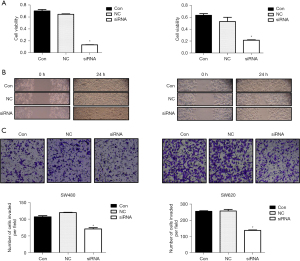
Suppression of RhoA/ROCK2 signaling pathway significantly inhibited the proliferation and invasion of colorectal cancer cell lines
To investigate the mechanism of RhoA/ROCK2 signaling pathway’s influence in colorectal cancer, we first demonstrated that the activation of RhoA/ROCK2 pathway could be significantly inhibited with Y-27632 (inhibitors of the RhoA/ROCK2 signaling pathway, 10 µmol/mL, 24 hours). Compared with normal control group and flank control group, the protein expressions of pMYPT1, cyclin D1 and MMP2 in Y-27632 group were significantly decreased (Figure 5A). Subsequently, CCK8 and Transwell assays were performed to detect the cell proliferation, metastasis, and invasion function of the normal control and Y-27632 groups. The results showed that RhoA/ROCK2 signaling pathway inhibitor could significantly inhibit the proliferation (Figure 5B), metastasis (Figure 5C), and invasion (Figure 5D) of colorectal cancer cell lines. These results suggest that RhoA/ROCK2 signaling pathway plays an important role in the progression of colorectal cancer and that activation of this pathway can promote tumor progression.
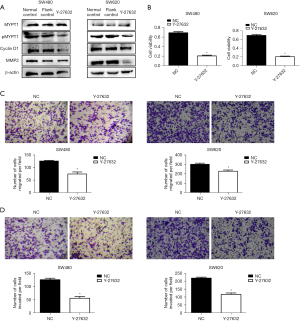
HIF-1α promoted the proliferation, migration, and invasion of colorectal cancer cell lines via RhoA/ROCK2 signaling pathway
In order to verify whether HIF-1α regulates colorectal progression through the RhoA/ROCK2 signaling pathway, colorectal cancer cell lines SW480 and SW620 were transfected with LV-RhoA lentivirus to overexpress RhoA, while the proliferation, migration, and invasion of the cells were detected with CCK8 and Transwell assays. In the SW480 cell line, cell viability was detected with CCK8 assay, and it was found that the cell viability of the siRNA + LV-RhoA group was significantly higher than that of the siRNA group (Figure 6A). Transwell assay was performed to detect the migration and invasion ability of cells, and similar results were obtained, with the migration and invasion ability of the siRNA + LV-RhoA group being significantly higher than that of siRNA group (Figure 6B,6C). In the SW620 cell line, we found that the cell viability of the siRNA + LV-RhoA group was significantly higher than that of the inhibitor Y-27632 group (Figure 6A). The Transwell assay produced similar results in terms of cell migration and invasion ability (Figure 6B,6C). The Western blot results showed that the expression levels of pMYPT1, cyclin D1, and MMP2 in the siRNA + LV-RhoA group were also significantly increased compared with those in the siRNA group (Figure 7). These results suggest that HIF-1α promotes the proliferation, migration, and invasion of colon cancer cell lines through RhoA/ROCK2 pathway.
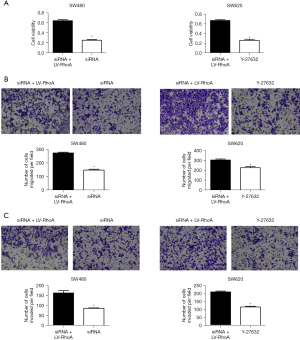
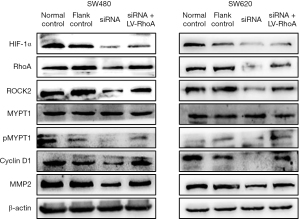
Discussion
Hypoxia is one of the most common features of the TME and has been shown to be associated with the progression and aggressiveness of malignant tumors (20). HIF-1α is a major regulator of hypoxic-induced cell biological responses and plays an important role in tumor-related neovascularization, proliferation, and apoptotic resistance (21). Tang et al. found that HIF-1α may act as a carcinogenic transcription factor in esophageal cancer by directly targeting TCF4/TCF7L2 and activating the Wnt/β-catenin pathway (22). A study has shown that hypoxia can not only directly affect tumor cells but can also enhance the invasive function of tumor cells by affecting extracellular matrix elements, such as fibroblasts (23). Multiple clinical studies have shown that HIF-1α is highly expressed in tumor tissues, such as those of colorectal cancer (13), breast cancer (24), pancreatic cancer (25), kidney cancer (26), ovarian cancer (27), and esophageal cancer (28). Although HIF-1α has been reported in colorectal cancer, its specific molecular regulatory mechanisms remain unclear. In this study, we collected clinical tissue samples and performed IHC, Western blotting, and PCR: consistent with previous studies, the protein expression level and mRNA expression level of HIF-1α in the colorectal cancer tissues we collected were significantly higher than those in normal tissues. To investigate the molecular mechanism of HIF-1α in colorectal cancer, we silenced HIF-1α expression with siRNA; subsequent CCK-8, Transwell, and wound healing assays revealed that silencing HIF-1α could significantly inhibit the proliferation and invasion of colorectal cancer cell lines. Hypoxia is an important part of the TME, and HIF-1α, as a hypoxia-inducing factor, plays an important role in tumor progression. Our study also indicates the important role of HIF-1α in the progression of colorectal cancer, providing an important theoretical basis for the treatment of colorectal cancer.
The inactivated form of RhoA is converted to RhoA-GTP and activates ROCK2 (MYPT-1 is a specific substrate, and the p-MYPT1/MYPT-1 ratio is an indicator of ROCK2 activity), which in turn induces the phosphorylation of MYPT1 and MLC (29). Previous studies have suggested that P2Y12R may be involved in neuropathic pain by regulating the activation of RhoA and ROCK (18,30). The spinal RhoA/ROCK2 pathway may be a key downstream target of CXCR4-mediated neuronal sensitization and hypersensitivity to pain in bone cancer, and may be an effective target for pain treatment (31). miR-451b regulated by transcription factor p300 may protect cigarette smoke-induced cellular stress by downregulating RhoA/ROCK2 pathway (32). However, the expression and mechanism of RhoA/ROCK2 pathway in colorectal cancer have not been examined. In this study, we found that the results of immunohistochemistry, Western blot, and PCR all showed that the protein expression levels and mRNA expression levels of RhoA and ROCK2 in colorectal cancer tissues were significantly higher than those in normal tissues, indicating that RhoA/ROCK2 pathway plays an important role in the occurrence and development of colorectal cancer.
The correlation between HIF-1α and RhoA/ROCK2 pathway and whether HIF-1α can regulate the activation of RhoA/ROCK2 pathway have not been extensively investigated. In order to determine the correlation between HIF-1α and RhoA/ROCK2 pathway, we first established the colocalization of HIF-1α and RhoA through immunofluorescence experiments, suggesting that there is a possible correlation between them. We then used siRNA to silence the expression of HIF-1α and found that the expression levels of RhoA/ROCK2 pathway-related proteins, pMYPT1, cyclin D1, and MMP2, were significantly reduced, suggesting that HIF-1α could regulate the activation of RhoA/ROCK2 pathway. Finally, in order to verify whether HIF-1α promotes the progression of colorectal cancer by regulating the activation of RhoA/ROCK2 pathway, we used siRNA to silence HIF-1α expression while using LV-RhoA to overexpress the RhoA, and RhoA/ROCK2 pathway inhibitor, Y-27632. Combined with the rescue experiment, the findings suggest that HIF-1α regulates the progression of colorectal cancer by regulating the RhoA/ROCK2 pathway. In summary, our study identifies a novel molecular regulatory mechanism in colorectal cancer progression, providing a new target for colorectal cancer patients.
Conclusions
Our study identified a hitherto undefined molecular mechanism of colorectal cancer and demonstrated that hypoxia plays an important role in the TME during the occurrence and development of colorectal cancer. HIF-1α promotes the progression of colorectal cancer by activating the RhoA/ROCK2 signaling pathway.
Acknowledgments
Funding: None.
Footnote
Reporting Checklist: The authors have completed the MDAR reporting checklist. Available at https://jgo.amegroups.com/article/view/10.21037/jgo-23-844/rc
Data Sharing Statement: Available at https://jgo.amegroups.com/article/view/10.21037/jgo-23-844/dss
Peer Review File: Available at https://jgo.amegroups.com/article/view/10.21037/jgo-23-844/prf
Conflicts of Interest: All authors have completed the ICMJE uniform disclosure form (available at https://jgo.amegroups.com/article/view/10.21037/jgo-23-844/coif). The authors have no conflicts of interest to declare.
Ethical Statement: The authors are accountable for all aspects of the work in ensuring that questions related to the accuracy or integrity of any part of the work are appropriately investigated and resolved. The study was conducted in accordance with the Declaration of Helsinki (as revised in 2013). The study was approved from the Ethics Committee of the First Affiliated Hospital of Chongqing Medical University (Chongqing, China) (Approval No. 20150612), and informed consent was taken from all the patients.
Open Access Statement: This is an Open Access article distributed in accordance with the Creative Commons Attribution-NonCommercial-NoDerivs 4.0 International License (CC BY-NC-ND 4.0), which permits the non-commercial replication and distribution of the article with the strict proviso that no changes or edits are made and the original work is properly cited (including links to both the formal publication through the relevant DOI and the license). See: https://creativecommons.org/licenses/by-nc-nd/4.0/.
References
- Cervantes A, Adam R, Roselló S, et al. Metastatic colorectal cancer: ESMO Clinical Practice Guideline for diagnosis, treatment and follow-up. Ann Oncol 2023;34:10-32. [Crossref] [PubMed]
- Arnold M, Sierra MS, Laversanne M, et al. Global patterns and trends in colorectal cancer incidence and mortality. Gut 2017;66:683-91. [Crossref] [PubMed]
- Davila RE, Rajan E, Baron TH, et al. ASGE guideline: colorectal cancer screening and surveillance. Gastrointest Endosc 2006;63:546-57. [Crossref] [PubMed]
- Semenza GL. Oxygen sensing, hypoxia-inducible factors, and disease pathophysiology. Annu Rev Pathol 2014;9:47-71. [Crossref] [PubMed]
- Fitzpatrick SF. Immunometabolism and Sepsis: A Role for HIF? Front Mol Biosci 2019;6:85. [Crossref] [PubMed]
- Li Y, Zhao L, Li XF. Hypoxia and the Tumor Microenvironment. Technol Cancer Res Treat 2021;20:15330338211036304. [Crossref] [PubMed]
- Singleton DC, Macann A, Wilson WR. Therapeutic targeting of the hypoxic tumour microenvironment. Nat Rev Clin Oncol 2021;18:751-72. [Crossref] [PubMed]
- Kunej T. Integrative Map of HIF1A Regulatory Elements and Variations. Genes (Basel) 2021;12:1526. [Crossref] [PubMed]
- Fratantonio D, Cimino F, Speciale A, et al. Need (more than) two to Tango: Multiple tools to adapt to changes in oxygen availability. Biofactors 2018;44:207-18. [Crossref] [PubMed]
- Koh MY, Powis G. Passing the baton: the HIF switch. Trends Biochem Sci 2012;37:364-72. [Crossref] [PubMed]
- Suzuki N, Gradin K, Poellinger L, et al. Regulation of hypoxia-inducible gene expression after HIF activation. Exp Cell Res 2017;356:182-6. [Crossref] [PubMed]
- Dzhalilova D, Makarova O. Differences in Tolerance to Hypoxia: Physiological, Biochemical, and Molecular-Biological Characteristics. Biomedicines 2020;8:428. [Crossref] [PubMed]
- Kaidi A, Williams AC, Paraskeva C. Interaction between beta-catenin and HIF-1 promotes cellular adaptation to hypoxia. Nat Cell Biol 2007;9:210-7. [Crossref] [PubMed]
- Gonzalez FJ, Xie C, Jiang C. The role of hypoxia-inducible factors in metabolic diseases. Nat Rev Endocrinol 2018;15:21-32. [Crossref] [PubMed]
- Chen H, Chen J, Yuan H, et al. Hypoxia-inducible factor-1α: A critical target for inhibiting the metastasis of hepatocellular carcinoma. Oncol Lett 2022;24:284. [Crossref] [PubMed]
- Hall A. Rho GTPases and the control of cell behaviour. Biochem Soc Trans 2005;33:891-5. [Crossref] [PubMed]
- Komagome R, Kimura K, Saito M. Postnatal changes in Rho and Rho-related proteins in the mouse brain. Jpn J Vet Res 2000;47:127-33. [PubMed]
- Tatsumi E, Yamanaka H, Kobayashi K, et al. RhoA/ROCK pathway mediates p38 MAPK activation and morphological changes downstream of P2Y12/13 receptors in spinal microglia in neuropathic pain. Glia 2015;63:216-28. [Crossref] [PubMed]
- Mottawea W, Chiang CK, Mühlbauer M, et al. Altered intestinal microbiota-host mitochondria crosstalk in new onset Crohn's disease. Nat Commun 2016;7:13419. [Crossref] [PubMed]
- Muz B, de la Puente P, Azab F, et al. The role of hypoxia in cancer progression, angiogenesis, metastasis, and resistance to therapy. Hypoxia (Auckl) 2015;3:83-92. [Crossref] [PubMed]
- Pouysségur J, Dayan F, Mazure NM. Hypoxia signalling in cancer and approaches to enforce tumour regression. Nature 2006;441:437-43. [Crossref] [PubMed]
- Tang K, Toyozumi T, Murakami K, et al. HIF-1α stimulates the progression of oesophageal squamous cell carcinoma by activating the Wnt/β-catenin signalling pathway. Br J Cancer 2022;127:474-87. [Crossref] [PubMed]
- Jing X, Yang F, Shao C, et al. Role of hypoxia in cancer therapy by regulating the tumor microenvironment. Mol Cancer 2019;18:157. [Crossref] [PubMed]
- Rezaeian AH, Li CF, Wu CY, et al. A hypoxia-responsive TRAF6-ATM-H2AX signalling axis promotes HIF1α activation, tumorigenesis and metastasis. Nat Cell Biol 2017;19:38-51. [Crossref] [PubMed]
- Liu M, Zhong J, Zeng Z, et al. Hypoxia-induced feedback of HIF-1α and lncRNA-CF129 contributes to pancreatic cancer progression through stabilization of p53 protein. Theranostics 2019;9:4795-810. [Crossref] [PubMed]
- Alsaab HO, Sau S, Alzhrani RM, et al. Tumor hypoxia directed multimodal nanotherapy for overcoming drug resistance in renal cell carcinoma and reprogramming macrophages. Biomaterials 2018;183:280-94. [Crossref] [PubMed]
- Kitajima S, Lee KL, Hikasa H, et al. Hypoxia-inducible factor-1α promotes cell survival during ammonia stress response in ovarian cancer stem-like cells. Oncotarget 2017;8:114481-94. [Crossref] [PubMed]
- Koukourakis MI, Giatromanolaki A, Skarlatos J, et al. Hypoxia inducible factor (HIF-1a and HIF-2a) expression in early esophageal cancer and response to photodynamic therapy and radiotherapy. Cancer Res 2001;61:1830-2. [PubMed]
- Aburima A, Wraith KS, Raslan Z, et al. cAMP signaling regulates platelet myosin light chain (MLC) phosphorylation and shape change through targeting the RhoA-Rho kinase-MLC phosphatase signaling pathway. Blood 2013;122:3533-45. [Crossref] [PubMed]
- Yu T, Zhang X, Shi H, et al. P2Y12 regulates microglia activation and excitatory synaptic transmission in spinal lamina II neurons during neuropathic pain in rodents. Cell Death Dis 2019;10:165. [Crossref] [PubMed]
- Xu H, Peng C, Chen XT, et al. Chemokine receptor CXCR4 activates the RhoA/ROCK2 pathway in spinal neurons that induces bone cancer pain. Mol Pain 2020;16:1744806920919568. [Crossref] [PubMed]
- Shen W, Wang S, Wang R, et al. Transcription Factor p300 Regulated miR-451b Weakens the Cigarette Smoke Extract-Induced Cellular Stress by Targeting RhoA/ROCK2 Signaling. Oxid Med Cell Longev 2022;2022:7056283. [Crossref] [PubMed]